Peritoneal dialysis (PD) is a safe and effective form of renal replacement therapy. However, there are complications that limit long-term technique survival. Among them we find peritonitis and peritoneal fibrosis. Both disorders are characterized by changes in cell number.
During acute peritonitis, there are sudden changes in peritoneal fluid cell number, as well as loss of mesothelial cells. Peritoneal fibrosis is characterized by loss of mesothelium, and increased number of fibroblasts and peritoneal vessels. Tissue cell number is carefully regulated through the balance between cell birth (mitosis) and cell death, with occasional participation of cell migration or cell transdifferentiation (Fig. 1)1,2. An imbalance between these processes can result in disorders of cell number characterized by an excessive (e.g. neoplasia or peritoneal neovascularization) or insufficient cell number (e.g. neurodegenerative diseases or peritoneal demesothelization).
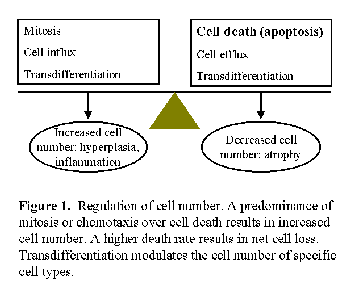
Apoptotic cell death is one of the key processes that regulate cell number. There is evidence that apoptosis participates in peritoneal injury. Stimuli that induce peritoneal cell apoptosis also induce peritoneal injury in vivo, apoptosis has been observed during peritoneal disease, the expression and/or activity of apoptosis modulatory molecules changes in the course of peritoneal disease and therapeutic maneuvers that target apoptosis improved the evolution of al least one complications of PD: peritonitis3.
Thus, understanding the contribution of apoptosis to peritoneal injury offers the opportunity to decrease the negative influence of the complications of the technique.
APOPTOSIS
Apoptosis is an active mode of cell death (cell suicide) under molecular control1. Apoptosis is characterized by morphological and functional changes (Fig 2).
Apoptosis is an essential process to remove unwanted and harmful cells and to maintain homeostasis of cell number. Teleologically apoptosis is an organized dismantling of cellular structures de×ed to limit tissue damage, while necrosis is an accidental cell death. The intensity of the lethal stimulus, the availability of ATP (energy) or the inactivation of caspases may determine whether the cell dies through apoptosis or necrosis1.
Tissues depend on well-ordered apoptosis and cell replacement for normal functioning. However, excessive, insufficient or untimely apoptosis may result in pathology. For this reason any therapeutic measure that interferes with apoptosis should be targeted as narrowly as possible to a single cell population.
The de× of appropriate therapeutic strategies requires a correct understanding of the role of apoptosis in peritoneal injury and of the molecular regulation of peritoneal cell apoptosis.
REGULATION OF APOPTOSIS
Cell death is usually a response to the cell microenvironment. The absence of certain factors (survival factors) or the presence of lethal factors promotes apoptosis. Surrounding cells synthesize survival or lethal factors or compete for such factors. A lethal cell microenvironment activates intracellular factors that promote apoptosis and/or inactivate intracellular survival factors. Known intracellular activators of apoptosis include death receptors, proapoptotic members of the Bcl2 family, endoplasmic reticulum stress and mitochondrial injury (Fig. 3). Caspases are a family of intracellular proteases that include both activators and effectors of apoptosis.
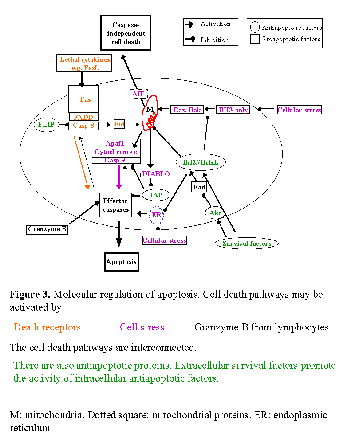
ROLE OF APOPTOSIS IN PD
Cell death through apoptosis has been documented in the course of peritoneal injury both in animal models and in clinical PD4-8. In addition, changes in the expression or activity of apoptosis regulatory factors have been observed in cultured peritoneal cells and in vivo peritoneal injury3.
However, few studies have addressed the role of apoptosis in PD by directly modulating its occurrence4. From information gathered in other tissue systems, different roles for apoptosis in peritoneal pathophysiology can be envisioned that depend on the cell type undergoing apoptosis and the amount and timing of apoptosis (Table 1). Apoptosis could be a cause of peritoneal damage, a mode of restoring normal tissue structure and a mechanism for persistence and progression of peritoneal injury.
Apoptosis may be beneficial if it occurs as a compensatory response to cellular proliferation or as a mode of eliminating irreversibly damaged cells. For example, apoptosis could contribute to resolution of fibrosis by eliminating excess fibroblasts, as demonstrated in the skin following the acute phase of scarring9, or could limit neovascularization10.
Under these circumstances, promotion of apoptosis in certain cell types might protect from chronic peritoneal changes associates with peritoneal dysfunction, such as fibrosis and increased vascularization. By contrast, induction of apoptosis by either exogenous factors, such as PD solutions, or endogenous mediators, could contribute to demesothelization both in the setting of acute peritonitis or chronic PD3.
Apoptosis also regulates inflammation and the immune response3, 11-15. Clearance of inflammatory cells by apoptosis contributes to resolution of inflammation. However, excessive or untimely apoptosis also contributes to an impaired host response in the setting of severe infection, such as sepsis12-14. The disposal of apoptotic cells also influences immunity and inflammation15.
From this brief glimpse at the diverse potential roles of apoptosis in peritoneal injury, we can infer that both the cell target and the timing of the intervention on apoptosis may have a re§able influence on the ultimate therapeutic consequences. It is necessary to better define the kinetics of apoptosis of different peritoneal cell populations in the course of peritoneal injury and repair.
PECULIARITIES OF PD
PD offers a unique window to access repetitively and unaggresively the protagonists of the inflammatory process in the human being. The daily disposal of peritoneal effluent allows the study of inflammatory cells and the measurement of soluble inflammatory mediators. From this point of view it is tempting to extrapolate data obtained in other systems to the PD situation However, PD has several peculiarities. Both cells and mediators are diluted several times a day by the PD exchange. In addition, the action takes paces in the presence of a uremic milieu and of poorly biocompatible PD solutions. Despite these caveats, data obtained in PD may help
unravel the contribution of apoptosis to inflammation and tissue injury in other settings.
PD SOLUTIONS AND THE MODULATION OF APOPTOSIS
There are several types of PD solutions, whose influence on peritoneal cell apoptosis may differ (Table 2). Published studies have only addressed the effects of a handful of these solutions, mostly in the cell culture and animal settings (Table 2).
PD solutions are cytotoxic to leukocytes and mesothelial cells. Several groups have addressed the mode of cell death (apoptosis versus necrosis). Three independent groups have tried to simulate in vivo conditions by exposing leukocytes to undiluted PD solutions for 10 to 30 minutes and then diluting the sample with spent dialysate or cell culture medium and studying apoptosis at 4 to 24h16-18. Traditional glucose-, high glucose degradation product (GDP)- containing PD solutions buffered with lactate accelerate neutrophil apoptosis in a caspase-dependent manner, especially when glucose concentration (and GDP content) was higher16.
As a consequence, the antibacterial function of these cells decreases16. The main proapoptotic component of PD solutions appears to be GDPs, as the toxicity could not be reproduced by adding exogenous glucose to lactate buffered solutions. In addition, glucose and low GDP solutions, such as Balance® and Bicavera® (Fresenius Medical Care, Bad Homburg, Germany) did not accelerate neutrophil apoptosis16 and unpublished observation).
Ongoing studies are characterizing the particular GDP responsible for this lethal effect. PD solutions also promote monocyte apoptosis17. A third group reported that high glucose, high GDP PD solutions induce monocyte necrosis. However, their techniques did not allow to fully discriminate between primary necrosis and late apoptosis18.
A higher lethality of high glucose, high GDP solutions, not present in high glucose, low GDP solutions has been observed by other authors19. In this case neutrophils were exposed to undiluted dialysate for hours. As a consequence necrosis was more prevalent. However the technique used to quantify cell death did not allow to discriminate late apoptosis from necrosis. These authors used electron microcopy to confirm the mode of cell death by morphological features. However, they did not report the results obtained with this technique in samples incubated with high glucose/high GDP PD solution19.
Glucose/GDP containing PD solutions also induce apoptosis in cultured mesothelial cells. This effect takes 48 to 72 h to develop20,21 and has been related to glucose/GDP content20 or calcium content21. A 1.36% glucose solution did not induce apoptosis at 16h22. The proapoptotic effect of PD solutions on mesothelial cells has been confirmed in
experimental models in vivo6-8.
PERITONITIS
Both apoptosis of mesothelial cells and leukocytes takes place during human PD peritonitis5 (Fig. 4). Apoptotic cells (mostly neutrophils) have also been documented in peritoneal effluents of mice with peritonitis in the presence of PD solutions4. The percentage of apoptotic cells is low in the early stages of peritonitis, both in the human and the experimental setting. However, given the high number of cells in these early effluents, the absolute number of apoptotic cells is high (around 175 apoptotic cells/µL in humans).
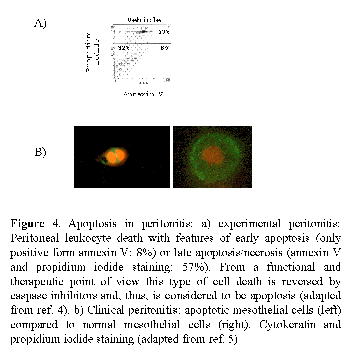
During the evolution of peritonitis, and coinciding with the decrease in total leukocyte number, the percentage of total apoptotic cells increases. In this setting, it has been suggested that mesothelial apoptosis is deleterious, as it may contribute to demesothelization. By contrast, neutrophils are programmed to die by apoptosis at the site of inflammation. This helps limit the inflammatory response. However, accelerated leukocyte apoptosis, such as that promoted by high glucose, high glucose degradation products (GDP) PD fluids or bacterial products may compromise the peritoneal defense.
Evidence to support this concept is derived from three
different experimental settings.
A) Maneuvers such as LPS priming result both in decreased neutrophil apoptosis and accelerated bacterial clearance in mice23.
B) High-glucose, GDP-containing PD solution accelerate neutrophil apoptosis in culture16 and
C) High-glucose, GDP-containing PD solution accelerate neutrophil apoptosis during experimental peritonitis4.
Accelerated neutrophil apoptosis in culture leads to decreased bacterial phagocytosis16. In vivo, accelerated neutrophil apoptosis is associated to a trend toward a slower bacterial clearance4. In both settings the pan-caspase inhibitor zVAD prevents neutrophil apoptosis and accelerates bacterial clearance, suggesting that the accelerated leukocyte apoptosis promoted by PD solutions impairs the peritoneal defense. The contribution of lymphocyte apoptosis to the peritoneal defense in PD has not been addressed yet. Contrary to neutrophils, inflammatory macrophages are able to recirculate and do not die locally24. However, PD solutions accelerate monocyte apoptosis in culture17 and their possible influence on macrophage apoptosis in vivo has not been addressed either.
During peritonitis there is another potential source of proapoptotic stimuli: lethal cytokines belonging to the TNF superfamily bind to and activate cell membrane death receptors25. TNF, FasL and TRAIL may be synthesized by infiltrating leukocytes and intrinsic peritoneal cells, including mesothelial cells5. FasL, but not TNF nor TRAIL, induces apoptosis of mesothelial cells from PD patients5, especially upon exposure to other inflammatory cytokines5.
PD peritonitis is usually a mild complication characterized by a rapid response to i.p. antibiotics. However, agents such as S. aureus or gram negative bacteria may cause severe and protracted peritonitis even when antibiotic treatment is optimized. For this reason a murine model of peritonitis induced by S. aureus was chosen to study the possible in vivo relevance of apoptosis in PD defense4. In the presence of high glucose, high GDP PD solutions the pan-caspase inhibitor zVAD increased the number of viable neutrophils and this was associated with an accelerated clearance of the bacteria.
These results are consistent with those reported in experimental models of septic polymicrobial peritonitis in the absence of PD solutions. In these models, prevention of apoptosis in other cell types may have contributed to the therapeutic effect of caspase inhibitors12-14. In this regard, maneuvers that prevent lymphocyte or epithelial cell apoptosis also improve the prognosis of septic peritonitis13,14. One unresolved issue is whether a prolonged survival or leukocytes, given the non-specific nature of the innate responses, may be detrimental to mesothelium. In this regard, mesothelium might also be protected be apoptosis inhibitors.
Once safe caspase inhibitors are available for their use in humans, trials should be de×ed to test whether their administration together with antibiotics can accelerate the recovery of the more severe cases of peritonitis.
ZVAD may also inhibit the processing of proinflammatory cytokines, such as IL-1 and IL-18, by caspases. The possible contribution of this effect to the therapeutic effect is unknown.
The fact that there were no differences in total leukocyte number between animals treated with zVAD or vehicle argues against this possibility4.
CHRONIC LOSS OF MESOTHELIUM
The contribution of apoptosis to the chronic loss of mesothelium upon exposure to PD solutions has been documented in experimental animals6-8. These data support the biological ×ificance of the in vitro observations on the toxicity of PD fluids20,21. High glucose PD solutions led to a decreased proliferation/apoptosis ratio in mesothelial cells, suggesting a disequilibrium favoring cell depletion6.
Chronic ip instillation of glucose solutions led to a ×ificant increase in the number of non-viable mesothelial and apoptotic cells8. The lethal effect was higher when lactate-based, high GDP PD solutions were used8. Chronic instillation of icodextrin also increases mesothelial cell apoptosis in rats7. However, mesothelial cell apoptosis in response to PD solutions has not been studied in human PD in vivo. In addition there is a total lack of information on apoptosis of other cell types in chronic PD and on the relationship of apoptosis to transdifferentiation2.
THERAPEUTIC APPROACHES
Understanding the role and regulation of apoptosis in peritoneal disease has the potential to provide the basis for the de× of new therapeutic strategies as well as to improve the biocompatibility of PD fluids. Among the cellular targets, we might be interested in prolonging mesothelial cell survival in chronic PD and during acute peritonitis. Conversely, fibroblasts or vessels may be targeted with proapoptotic maneuvers, in order to limit fibrosis and excessive peritoneal vascularization.
The key to any successful therapeutic manipulation of apoptosis lies in limiting the interference to the cell type we want to manipulate and to a defined time period. Otherwise, we risk unintended side effects derived from interference with physiological apoptosis taking place during peritoneal healing or in the every day function of other organs. For instance, a theoretical risk of promoting neoplasia can be predicted if there are no temporal limits to antagonism of apoptosis. Mutations in apoptosis regulatory proteins that favor cell survival are common in neoplasias.
There are different theoretical approaches to achieve cell specificity. The ideal one would be to define molecular pathways that are activated only under pathological conditions.
However, there is not enough information on this subject. Another would be a cell specific delivery of the drug. Gene therapy using cell specific promoters holds promise in this regard.
Finally if cell-specificity is not achieved, we might choose a non-specific inhibitor of apoptosis administered for a limited period of time. This limited period of time would be chosen according to the time-frame of maximal apoptosis in the target cell population. The fact that the peritoneum is directly accessible during PD can also be used to our advantage. Direct i.p. administration can be used as a means of drug delivery.
Acknowledgments: The author's research has been financed by grants from FIS 98/0637 and 01/0199, Fundación Conchita Rábago, Comunidad de Madrid (08.2/0030/2000),
Sociedad Española de Nefrología, Fresenius Medical Care and EU project QLG1-CT-2002-01215.
References
1. Ortiz A. Nephrology Forum: Apoptotic regulatory proteins in renal injury. Kidney Int 58:467-485, 2000
2. Yanez-Mo M, Lara-Pezzi E, Selgas R, Ramirez-Huesca M, Dominguez-Jimenez C, Jimenez-Heffernan JA, Aguilera A, Sanchez-Tomero JA, Bajo MA, Alvarez V, Castro MA, del Peso G, Cirujeda A, Gamallo C, Sanchez-Madrid F, Lopez-Cabrera M. Peritoneal dialysis and epithelial-to-mesenchymal transition of mesothelial cells. N Engl J Med 348:403-13, 2003
3. Ortiz A, Catalan MP. Will cell death modulation increase PD technique survival?. Perit Dial Int 2003 (accepted)
4. Catalán MP, Esteban J, Subirá D, Egido J, Ortiz A. Inhibition of caspases improves bacterial clearance in experimental peritonitis Perit Dial Int 23:123-126,2003
5. Catalan MP, Subirá D, Reyero A, Selgas R, Ortiz A, Egido J, Ortiz A. Regulation of apoptosis by lethal cytokines in human mesothelial cells. Kidney Int 64:321-330, 2003
6. Zheng Z, Ye R, Yu X, Bergstrom J, Lindholm B. Peritoneal dialysis solutions disturb the balance of apoptosis and proliferation of peritoneal cells in chronic dialysis model. Adv Perit Dial 17:53-7, 2001
7. Gotloib L, Wajsbrot V, Shostak A. Mesothelial dysplastic changes and lipid peroxidation induced by 7.5% icodextrin. Nephron 92:142-55, 2002
8. Gotloib L, Shostak A, Wajsbrot V, Kushnier R. High glucose induces a hypertrophic, senescent mesothelial cell phenotype after long in vivo exposure. Nephron 82:164-73, 1999
9. Desmouliere A, Redard M, Darby I, Gabbiani G. Apoptosis mediates the decrease in cellularity during the transition between granulation tissue and scar. Am J Pathol 146:56-66, 1995
10. Chavakis E, Dimmeler S. Regulation of endothelial cell survival and apoptosis during angiogenesis. Arterioscler Thromb Vasc Biol 22:887-93, 2002
11. Rathmell JC, Thompson CB. Pathways of apoptosis in lymphocyte development, homeostasis, and disease. Cell 109 Suppl:S97-107,2002
12. Hotchkiss RS, Tinsley KW, Swanson PE, Chang KC, Cobb JP, Buchman TG, Korsmeyer SJ, Karl IE. Prevention of lymphocyte cell death in sepsis improves survival in mice. Proc Natl Acad Sci USA 96:14541-6, 1999
13. Hotchkiss RS, Chang KC, Swanson PE, Tinsley KW, Hui JJ, Klender P, Xanthoudakis S, Roy S, Black C, Grimm E, Aspiotis R, Han Y, Nicholson DW, Karl IE. Caspase inhibitors improve survival in sepsis: a critical role of the lymphocyte. Nat Immunol 1:496-501, 2000
14. Coopersmith CM, Chang KC, Swanson PE, Tinsley KW, Stromberg PE, Buchman TG, Karl IE, Hotchkiss RS. Overexpression of Bcl-2 in the intestinal epithelium improves survival in septic mice. Crit Care Med 30:195-201, 2002
15. Savill J, Dransfield I, Gregory C, Haslett C. A blast from the past: clearance of apoptotic cells regulates immune responses. Nat Rev Immunol 2:965-75, 2002
16. Catalán MP, Reyero A, Egido J, Ortiz A. Acceleration of neutrophil apoptosis by glucose-containing peritoneal dialysis solutions: role of caspases. J Am Soc Nephrol 12:2442-9,2001
17. Jin HM, Di YD, Xu QJ. Effects of commercial glucose-based peritoneal dialysates on peripheral blood phagocytes apoptosis. Perit Dial Int 19 Suppl 2:S388-93, 1999
18. Plum J, Lordnejad MR, Grabensee B. Effect of alternative peritoneal dialysis solutions on cell viability, apoptosis/necrosis and cytokine expression in human monocytes. Kidney Int 54:224-35, 1998
19. Cendoroglo M, Sundaram S, Groves C, Ucci AA, Jaber BL, Pereira BJ. Necrosis and apoptosis of polymorphonuclear cells exposed to peritoneal dialysis fluids in vitro. Kidney Int 52:1626-34, 1997
20. Zheng ZH, Ye RG, Bergstrom J, Lindholm B. Effect of dialysate composition on the apoptosis and proliferation of human peritoneal mesothelial cells and protein expression of Fas and c-Myc. Adv Perit Dial 16:31-5, 2000
21. Yang AH, Chen JY, Lin YP, Huang TP, Wu CW. Peritoneal dialysis solution induces apoptosis of mesothelial cells. Kidney Int 51:1280-8, 1997
22. Plum J, Razeghi P, Lordnejad RM, Perniok A, Fleisch M, Fussholler A, Schneider M, Grabensee B. Peritoneal dialysis fluids with a physiologic pH based on either lactate or bicarbonate buffer-effects on human mesothelial cells. Am J Kidney Dis 38:867-75, 2001
23. Feterowski C, Weighardt H, Emmanuilidis K, Hartung T, Holzmann B. Immune protection against septic peritonitis in endotoxin-primed mice is related to reduced neutrophil apoptosis. Eur J Immunol 31:1268-77, 2001
24. Bellingan GJ, Caldwell H, Howie SE, Dransfield I, Haslett C. In vivo fate of the inflammatory macrophage during the resolution of inflammation: inflammatory macrophages do not die locally, but emigrate to the draining lymph nodes. J Immunol 157:2577-85, 1996
25. Ortiz A, Lorz C, Egido J. New kids in the block: the role of FasL and Fas in kidney damage. J Nephrol 12:150-158, 1999